Hydrogen
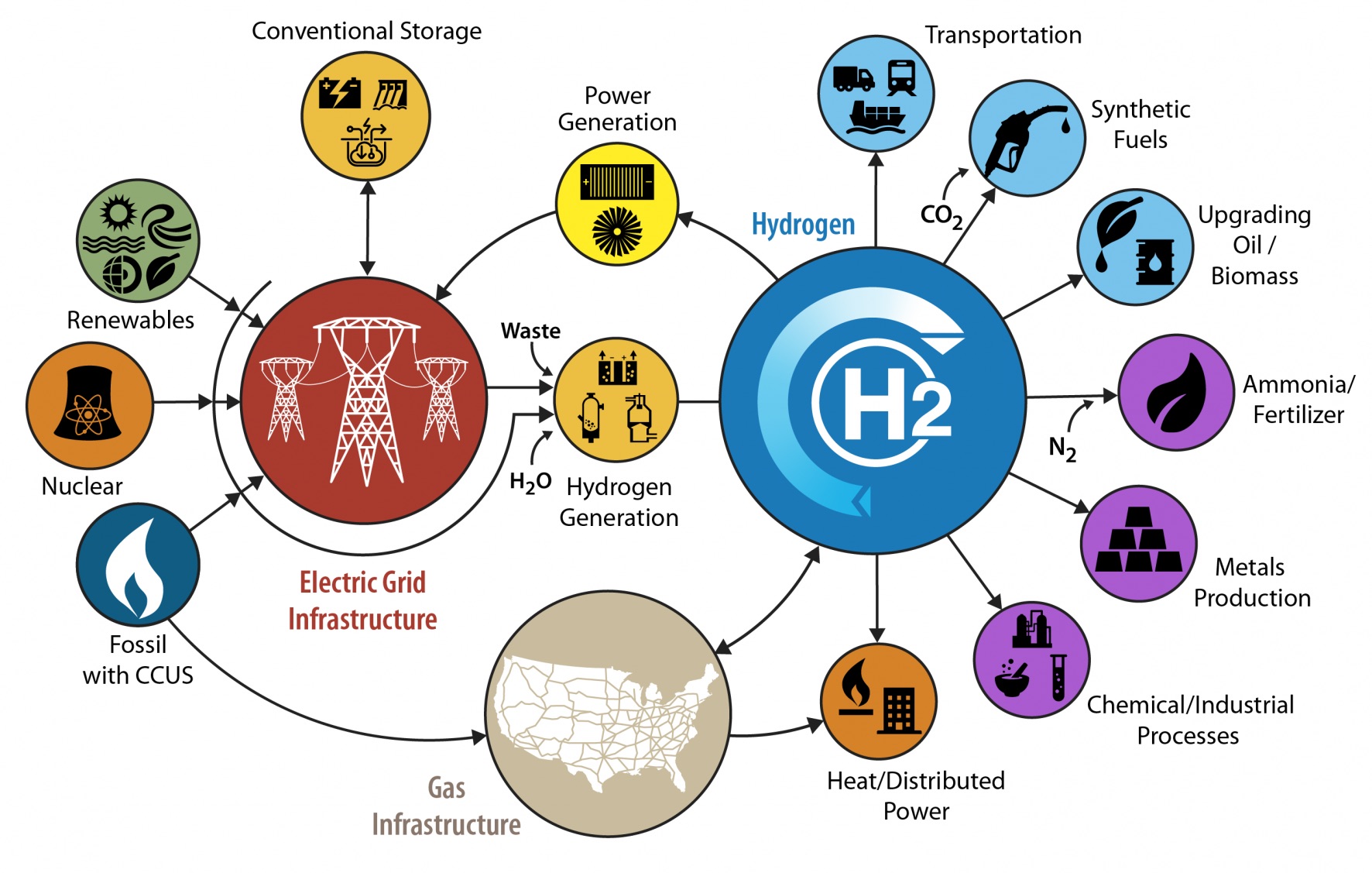
Hydrogen (Hâ‚‚) offers the potential for a transportable, storable fuel for a low-carbon economy. Hydrogen can be generated renewably using electrolysis and from natural gas, which when combined with carbon capture and storage can reduce greenhouse gas emissions. Current global hydrogen demand is 330 million metric tons of oil equivalent annually (roughly 6.5 million barrels of oil equivalent per day), primarily as a feedstock for industrial manufacturing purposes. The U.S. Department of Energy estimates that 1 percent of 2020 U.S. hydrogen production is from electrolysis, and 99 percent is from fossil fuels in the form of natural gas through steam reforming of methane (95 percent), and partial oxidation from coal gasification (4 percent). Fossil fuels are expected to continue as the main source of hydrogen through 2050 based on International Energy Agency projections driven by abundant supply, low cost, and expected development of large-scale carbon capture and storage. Regardless of the source of hydrogen, its use as an energy source at urban, regional, or national scale will require development of a robust supply network integrating storage, transportation, and distribution infrastructure. In the United States, an extensive natural gas pipeline network provides an excellent starting point for considering gas transportation and distribution options. However, current storage capacity for hydrogen is inadequate to allow for supply beyond industrial usage.
Storage
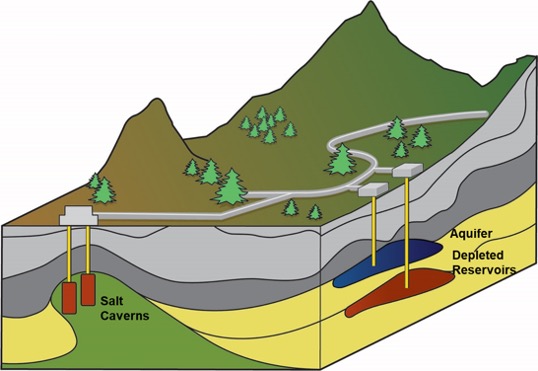
Geological storage combined with a hydrogen pipeline and transportation infrastructure connecting supply to end users will be necessary for development of a lower greenhouse gas emissions economy including hydrogen. At present in the United States, three subsurface hydrogen storage facilities have capacity for industrial usage. All three are located in salt caverns in southeastern Texas along the Gulf Coast. Salt caverns present the advantage of allowing fast withdrawal and injection rates but are expensive to create, of limited capacity, and restricted to areas with salt at the right depth. Porous-media (e.g., sandstone) reservoirs offer larger capacity and more-abundant opportunities for storage. They fall into two broad categories: (1) depleted oil and gas reservoirs, with proven storage volume, flow properties, and upper-seal capabilities; and (2) saline aquifers, with abundant potential locations but unproven gas-trapping and recovery characteristics. Depleted oil and gas reservoirs, particularly gas reservoirs, may also be advantaged by having basic infrastructure (for example, roads, production pads, pipelines, and wells) that may be partially repurposed for hydrogen storage and production. Other options, such as abandoned hard-rock mines, could be considered, but integrity for gas storage at pressure would be a major concern.
Although types of geological storage for hydrogen were assessed and modeled for meeting indicative urban transportation hydrogen demands (Lord and others, 2014), an inventory of actual sites needs to be created and evaluated with respect to hydrogen storage feasibility. We see a need to integrate the evaluation of specific potential storage sites with assessment of available gas pipeline networks to inform robust location-specific models and scenarios, which could be used to define road maps for hydrogen utilization for a broad range of energy uses, such as road or marine transport, electricity generation, and manufacturing.
Generation from In Situ Combustion
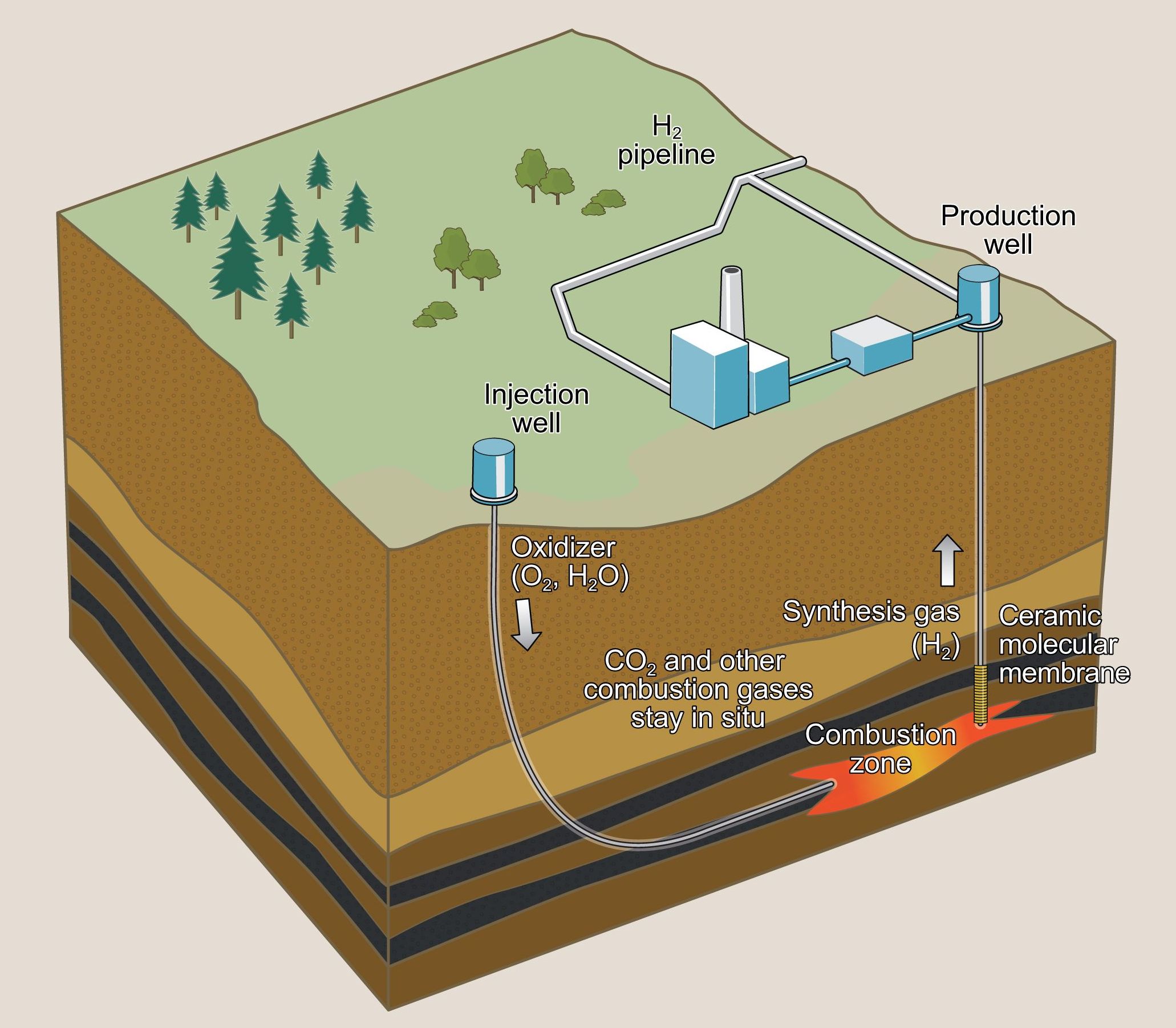
Hydrogen can be generated from in situ combustion (ISC) of hydrocarbons as well as from underground coal gasification (UCG) under controlled conditions. Pilot ISC (Cold Lake field, Canada) and multiple UCG projects in the 1970s and 1980s generated hydrogen in situ and produced the gas to the surface. However, investigation into hydrogen generation from in situ means did not receive much attention at the time. Continued research and applications of ISC for enhanced oil recovery has demonstrated the viability of this process for increased oil recovery in different reservoir types. We see an opportunity to investigate the potential of ISC for the primary purpose of hydrogen generation in porous media. The benefit of generating hydrogen from the subsurface is that it would provide a direct source of hydrogen utilizing thermal energy from combustion of hydrocarbons in place. If hydrogen can be produced at the wellhead from ISC, then carbon dioxide and other emissions can be separated and reinjected into the subsurface. New developments in selective-filtering membrane technology have the potential to be adapted for use downhole, opening the door for systems to allow for hydrogen production from the reservoir while retaining some or all of the cogenerated carbon dioxide and other emissions in the reservoir.
We envision a new and exciting area of research to investigate ISC for hydrogen production. The research pathway would start with reservoir and ISC modeling coupled with laboratory experiments to understand the reactions in hydrocarbon-filled porous media under different conditions. From that work, we would identify reservoir types and fields suitable for designing and conducting pilot tests leading to potential development. In parallel, work with materials scientists and engineers would be conducted to adapt and test ceramic molecular membranes for downhole hydrogen separation.
Economics – Hydrogen Value Chain
Scaling up the hydrogen sector requires a dedicated and developed transportation and storage infrastructure system. We recognize two gaps in our understanding of hydrogen's economic potential as part of the energy system.
First, while it is important to drill deep on technology solutions and application segments to resolve technical challenges, technical and foundational research only provides essential building blocks. It is paramount to develop a system-level understanding to inform consideration of options for the fast-emerging value chain. Technology options for hydrogen supply, demand, transportation, and storage need to be considered as a portfolio of solutions.
Second, the hydrogen value chain cannot be considered a stand-alone system. Instead, it must connect and interact with the existing system, which includes natural gas, renewables, downstream markets, etc. Currently, no market study or modeling system exists that can readily assess and analyze the interaction of hydrogen with the natural gas value chain, including power utilities as a traditional downstream segment.
Hence we focus on research to model the energy-economic system of the hydrogen value chain, assessing the cost competitiveness of various pathways and technology options to bring hydrogen to market, and identifying the optimal technology mix and routes to market.
Geology Matters
Whether considering subsurface options for hydrogen storage or investigating the potential for in situ generation of hydrogen from oil or gas fields, subsurface geological characterization and modeling are paramount. The Bureau of Economic Geology at The University of Texas is extremely well positioned, with subsurface-data access, numerical modeling capability, and laboratories to investigate interactions of rock and hydrogen in the subsurface, model hydrogen storage and supply economics, and investigate ISC processes to evaluate in situ hydrogen generation. The Bureau is also experienced in leading collaborative, multidisciplinary applied research efforts. We see an exciting opportunity to bring together researchers in geology, numerical modeling, chemical engineering, petroleum engineering, and materials science to generate integrated and innovative approaches for unlocking hydrogen as part of a low-emissions future.
Reference
Lord, A. S., Kobos, P. H., and Borns, D. J., 2014, Geologic storage of hydrogen: scaling up to meet city transportation demands: International Journal of Hydrogen Energy, v. 39, no. 28, p. 15570–15582.