Rock-Fabric
Classification
The classification
used here has been presented by Lucia (1983, 1995, 1999). The foundation
of the Lucia classification is the concept that pore-size distribution
controls permeability
and saturation
and that pore-size distribution is related to rock
fabric. Lucia (1983) showed that the most useful method of characterizing
rock fabrics petrophysically is to divide pore types into pore space
located between grains and crystals, called interparticle porosity,
and all other pore space, called vuggy porosity (Fig. 2). Interparticle
porosity is defined as pore space located between grains or crystals
and is not significantly larger that than the particles. Vuggy porosity
is pore space that is within grains or crystals or that is significantly
larger than grains or crystals; that is pore space that is not interparticle.
Vuggy pore space is further subdivided into two groups based on
how the vugs are interconnected; (1) vugs that are interconnected
only through the interparticle pore network are termed separate
vugs and (2) vugs that form an interconnected pore system are
termed touching
vugs. Vugs are commonly present as dissolved grains, fossil
chambers, fractures, and large irregular cavities. Although fractures
may not be formed by depositional or diagenetic processes, fracture
porosity is included because it defines a unique type of porosity
in carbonate reservoir rocks.
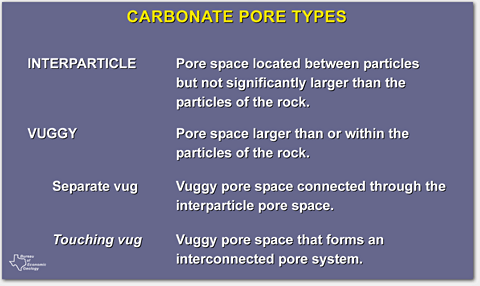 |
Figure
2. Petrophysical classification of carbonate pore space. |
In order to
relate carbonate rock
fabrics to pore-size distribution, it is important to 1) determine
which of the three major pore-type classes are present, interparticle,
separate-vug,
or touching-vug,
2) measure the volume (porosity) of interparticle and separate-vug
pore space, and 3) characterize the rock fabric of each pore type
petrophysically.
Classification
of Interparticle Pore Space
Interparticle
porosity is defined as pore space located between grains or crystals
that is not significantly larger than the particles (generally <2
x particle size). The pore-size distribution can be described in
terms of particle size, sorting and interparticle porosity
(Fig. 3). The volume of interparticle pore space is important because
it relates to pore-size distribution.
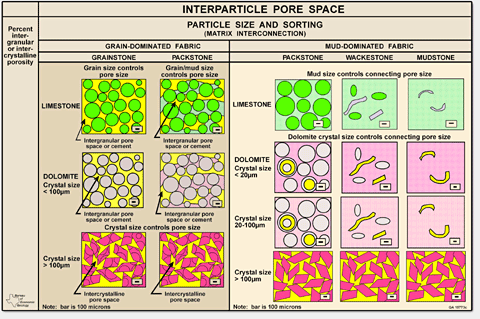 |
Figure
3. Geological/petrophysical classification of carbonate
interparticle pore space based on size and sorting of grains
and crystals. The volume of interparticle pore space is important
because it relates to pore-size distribution. |
Lucia (1983)
showed that particle size can be related to mercury capillary displacement
pressure in nonvuggy carbonates with more than 0.1 md permeability,
suggesting that particle size describes the size of the largest
pores (Fig. 4). Whereas the displacement pressure characterizes
the largest pore sizes and is largely independent of porosity,
the shape of the capillary
pressure curve characterizes the smaller pore sizes and is dependent
on interparticle porosity (Lucia 1983
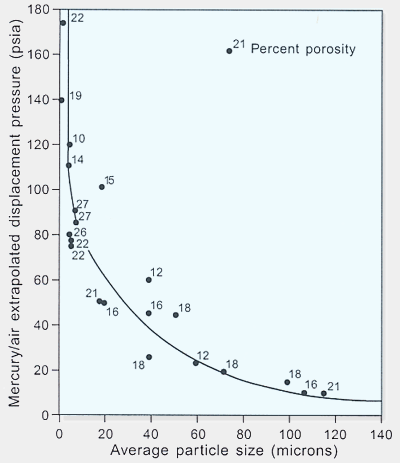 |
Figure
4. Relationship between mercury displacement pressure and average
particle size for nonvuggy carbonate rocks with permeability
greater than 0.1 md (Lucia, 1983). The displacement pressure
is determined by extrapolating the capillary
pressure curve to a mercury saturation
of zero. |
The relationship
between displacement
pressure and particle size (Fig. 4) is hyperbolic and suggests
important particle-size boundaries at 100 and 20 m. Lucia (1983)
demonstrated that three permeability
fields can be defined using particle-size boundaries of 100 and
20 ,
a relationship that appears to be limited to particle sizes of less
than 500 m (Fig. 5). Lucia (1995) named these three fields as petrophysical
class 1 (>100 )
class 2 (100-20 ),
and class 3 (< 20 ).
These class designations will be used throughout this presentation.
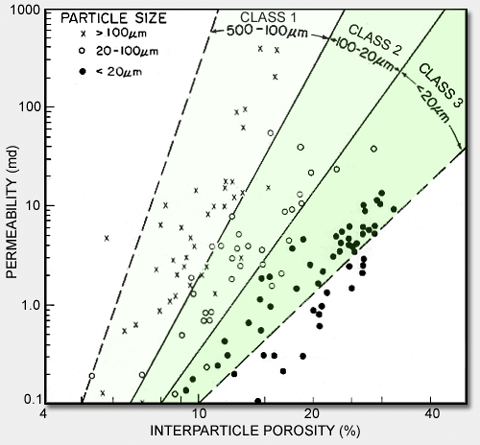 |
Figure
5. Porosity-air permeability relationship for various particle-size
groups in nonvuggy carbonate rocks (Lucia, 1983).
|
Recent work
has shown that permeability
classes can be better described in geologic terms if sorting as
well as particle size is considered. The approach to size and sorting
used in this petrophysical classification is similar to the grain-/mud-support
principle upon which the Dunham's (1962) classification is built.
Dunham's classification, however, is focused on depositional texture
whereas petrophysical
classifications are focused on contemporary rock
fabrics which include depositional and diagenetic textures.
Therefore, minor modifications must be made in Dunham's classification
before it can be applied to a petrophysical classification.
Instead of dividing
fabrics into grain support and mud support as in Dunham's classification,
fabrics are divided into grain-dominated and mud-dominated (Fig.
3). The important attributes of grain-dominated
fabrics are the presence of open or occluded intergrain porosity
and a grain-supported texture. The important attribute of mud-dominated
fabrics is that the areas between the grains are filled with
mud even if the grains appear to form a supporting framework.
Grainstone
is clearly a grain-dominated
fabric, but Dunham's packstone class bridges a boundary between
large intergrain pores in grainstone and small interparticle pores
in wackestones
and mudstones.
Some packstones have intergrain pore space and some have the intergrain
space filled with mud. The packstone textural class must be divided
into two rock-fabric
classes: grain-dominated packstones
that have intergrain pore space or cement and mud-dominated
packstones that have intergrain spaces filled with mud (Fig.
3).
Grainstone
= grain supported with no intergrain lime mud present.
Grain-dominated packstone = grain
supported with both intergrain pore space and lime mud.
Mud-dominated packstone = grain supported
with intergrain volume filled with lime mud.
Wackestone = mud supported with more
the 10 percent grains.
Mudstone = mud supported with less
than 10 percent grains.
|